
Affiliate of the Duke Regeneration Center
matthew.hilton@duke.edu
T: (919) 613-9761
Home
The Hilton Laboratory studies the cellular and molecular mechanisms underlying musculoskeletal development, disease, and regeneration. It is affiliated with the Departments of Orthopaedic Surgery and Cell Biology as part of the Duke Orthopaedic Cellular, Developmental, and Genome Laboratories within the Duke University School of Medicine.
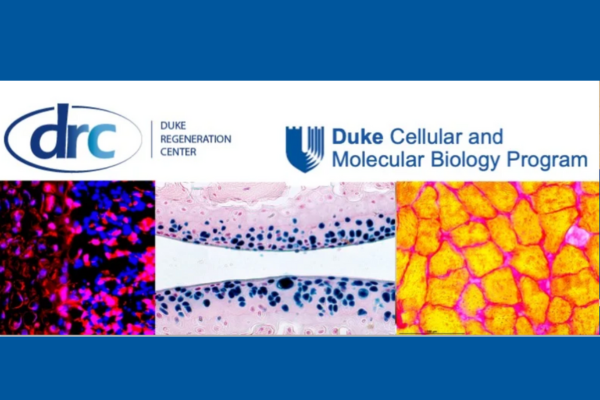
Research
The Hilton Lab research interests are aimed at uncovering the molecular circuitry regulating lineage commitment, proliferation, and differentiation of skeletal stem and progenitor cells (SSPCs), chondrocytes, osteoblasts, myoblasts, and non-myogenic mesenchymal cells within skeletal muscle, some of which are known as fibro-adipogenic progenitors (FAPs). My laboratory utilizes intricate surgical and genetic mouse models, biochemical approaches, and primary tissue/cell culture techniques to investigate the underlying tissue, cellular, and molecular mechanisms responsible for musculoskeletal development, disease, and regeneration. My lab has been mainly focused on the role of cell signaling mechanisms that influence these processes in both cell-autonomous and non-autonomous manners.
Musculoskeletal Development
Cellular signaling pathways are required during normal muscle, bone, and cartilage development—deregulation of these signaling circuits results in various skeletal dysplasias, muscular dystrophies, and other muscle disorders. We aim to understand the signaling mechanisms that regulate cell and stem cell proliferation, differentiation, fate determination, and self-renewal during musculoskeletal development using sophisticated mouse genetic approaches and complex cellular and molecular techniques.
Musculoskeletal Diseases
We study various musculoskeletal diseases, including osteoarthritis (OA), osteoporosis, and muscular dystrophy. Our laboratory has a particular interest in the genetics that underlie normal maintenance and pathological joint degeneration and pain associated with OA, the skeletal stem and progenitor cell (SSPC) dysregulation that leads to osteoporosis and low bone mass, as well as the dysfunction of non-myogenic mesenchymal cells implicated in muscle disease. We employ genetic and surgical mouse models and sophisticated cell and molecular analyses to understand the pathologies associated with these musculoskeletal diseases.
Musculoskeletal Regeneration
Damaged tissue from musculoskeletal injuries, such as bone fractures, critical-sized bone defects, or rotator cuff tears, often makes some attempt to repair or regenerate via mechanisms that are similar to processes involved in musculoskeletal development. Our laboratory is interested in uncovering the role that various cell or stem cell populations and signaling pathways play in musculoskeletal tissue regeneration. We are using genetic, surgical, and molecular approaches to understand the processes involved in musculoskeletal regeneration while developing approaches to enhance or accelerate musculoskeletal regeneration.
______________________________
Musculoskeletal Tissue Analytics Core
The Hilton Laboratory manages the Duke Musculoskeletal Tissue Analytics Core (MTAC), a unifying musculoskeletal tissue analysis resource designed to improve efficiency, accelerate research, innovate state-of-the-art procedures, and facilitate collaboration within the Duke University musculoskeletal research community. The MTAC provides services and mentoring/training opportunities in the areas of musculoskeletal whole tissue staining, x-ray, micro-computed tomography (µCT), histological, histomorphometric, and molecular analyses, including the performance and assessments of immunohistochemistry (IHC), immunofluorescence (IF), and in situ hybridization (ISH) on musculoskeletal tissue sections. The MTAC also develops innovative techniques that is transforming our ability to understand the interplay within and between bone, cartilage, muscle, and other tissues/cells during musculoskeletal development, disease, and regeneration.
People
The Hilton Laboratory is headed by Matthew J. Hilton, PhD. It is managed by Anthony J. Mirando, MS. The rest of the laboratory comprises research faculty, post-doctoral researchers, Duke University graduate students, and Duke University medical residents in the Department of Orthopaedic Surgery. If you are interested in joining the lab as a post-doc, graduate student, or medical resident, please contact us with your CV and a statement of research interests.
Matthew J. Hilton, PhD |
Anthony J. Mirando, MS |
Juliana Ibarra |
Helen Rueckert |
Publications
Selected Publications
|
This publication demonstrates that genetic ablation of Interleukin-6 (IL-6) alleviates both the cartilage degeneration and pain associated with post-traumatic osteoarthritis (PTOA); however, it does so in a sex (male) specific manner. This work identifies IL-6 and downstream effectors such as the Janus Kinases (JAKs), Signal Transducer and Activator of Transcription 3 (STAT3), and the Extracellular Signal-Regulated Kinases (ERK1/2) as specific mediators of PTOA-associated cartilage degradation and pain. These findings have significant implications for IL-6 as a common culprit and potential comprehensive (pain and cartilage degeneration) target as a DMOAD therapy while also being one of the first reports to detail sex-specific mechanisms underlying the pathobiology of PTOA. Science Signaling also highlighted this work with a “Focus” commentary, “Osteoarthritis and chronic pain: Interleukin-6 as a common denominator and therapeutic target”. Cover Image. It is also the most recent publication in a series of collaborative studies between my lab and Dr. Ru-Rong Ji (Duke Center of Translational Pain Medicine) on identifying molecular mediators of chronic musculoskeletal pain. |
This publication was the first to identify several non-myogenic mesenchymal cell populations localized within skeletal muscle tissue with specific differentiation capacities, unique responses to chronic and acute muscle injuries, and distinct localizations, including perineural and perivascular niches. Detailed characterizations and the development of new tools to identify, sort, and genetically target these populations have provided a foundation for future research into the behavior and function of these cells during skeletal muscle development, injury, and regeneration, as well as in neuromuscular diseases, such as the muscular dystrophies. This study is the inaugural publication representing a new transition for some of my research focus into skeletal muscle development, regeneration, and disease. |
|
This publication was the first to demonstrate that hypertrophic chondrocytes of the cartilage growth plate alter their cell fate by first dedifferentiating into populations of SSPCs, one of which is known as CXCL12-abundant reticular (CAR) cells, before their redifferentiation into osteoblasts (bone cells) and adipocytes (fat cells) within the bone marrow. This represents a paradigm shift away from our prior understanding of hypertrophic chondrocytes, one in which hypertrophic chondrocytes only indirectly supported endochondral bone formation by secreting factors that promote bone marrow vascularization, osteoblast recruitment and bone formation before undergoing apoptosis to leave behind an extracellular matrix scaffold utilized by the migrating osteoblasts. |
|
Utilizing genetic and molecular approaches, in this publication, we identified HES1 as a key transcriptional regulator downstream of Sonic Hedgehog (SHH/GLI) signaling capable of inducing preaxial polydactyly (PPD; extra fingers and toes), required for Gli3-deficient PPD, and sufficient to compensate for the digit number constraints of Shh-deficiency. While several SHH/GLI3-regulated genes in this setting have been identified, few have been directly implicated as critical regulators of SHH/GLI3-mediated digit development. This is especially true for target genes that don’t simply “feed-back” to regulate Shh expression, and no factors had been identified that were sufficient to compensate for Shh-deficiency. We view these findings as landmark discoveries in skeletal development and limb patterning. |
|
This publication is the first to describe precisely how specific HES factors regulate cartilage development. We developed unique in vivo genetic models and in vitro approaches to demonstrate that the RBPjκ-dependent Notch targets HES1 and HES5 suppress chondrogenesis and promote the onset of chondrocyte hypertrophy. HES1 and HES5 might have some overlapping functions in these processes, although only HES5 directly regulates Sox9 transcription to coordinate cartilage development. HEY1 and HEYL play no discernable role in regulating chondrogenesis or chondrocyte hypertrophy. In contrast, none of the HES or HEY factors appear to mediate Notch regulation of cartilage matrix catabolism during development. This work identifies important candidates that might function as downstream mediators of Notch signaling during normal skeletal development and Notch-related skeletal disorders. |
|
This publication is the first to describe how NOTCH signaling within bone marrow stromal/stem cells (BMSCs) and BMSCs are critical for routine fracture healing. Our data demonstrate that if NOTCH signaling is defective, specifically within BMSCs, the BMSC population is depleted and/or defective, resulting in severely compromised healing and fracture nonunion. Finally, our NOTCH defective BMSC animal model demonstrated an ability to produce a fracture nonunion irrespective of fracture stability and without any signs of altered vascular involvement. These are two fundamental and common causes of fracture nonunion. These data suggest that NOTCH signaling status within BMSCs and BMSC populations themselves could be predictors for those fractures that heal successfully and those that proceed to a nonunion. |
|
This publication describes how NOTCH signaling strength, duration, and context in joint cartilages mediate the balance between joint maintenance and osteoarthritis. We demonstrate that transient activation of NOTCH signaling in the joint cartilages favors normal physiology and maintenance and that sustained or pathological NOTCH signaling can induce osteoarthritis, independent of other external factors. This study is the first to reconcile how NOTCH signaling is required for joint maintenance and can induce joint disease. Furthermore, this work identified novel targets of the NOTCH signaling pathway in chondrocytes that mediate some of the destructive effects of sustained NOTCH signaling on joint tissues and has therefore implicated these molecules as potential disease-modifying osteoarthritis drug (DMOAD) targets. |
|
This publication describes the molecular mechanism by which the RBPjk-dependent Notch signaling pathway controls the onset of chondrocyte hypertrophy. We demonstrate that RBPjk-dependent Notch signaling indirectly suppresses Sox9 expression via secondary effectors, likely members of the HES/HEY transcription factor family. Furthermore, we demonstrate that this regulation coordinates the onset of chondrocyte maturation but has no effect on terminal chondrocyte hypertrophy and cartilage matrix turnover. |
|
Transient Gamma-Secretase Inhibition Accelerates and Enhances Fracture Repair Likely Via Notch Signaling Modulation. This publication describes a novel therapeutic strategy for accelerating and enhancing skeletal fracture repair. We demonstrate that transient, but not sustained, gamma-secretase and Notch inhibition promotes and accelerates fracture repair via the enhanced differentiation of skeletal progenitors. This study is the first to demonstrate that the gamma-secretase and Notch inhibitor, DAPT, can be utilized in skeletal regeneration and repair and serves as a proof-of-concept for further exploration of these drugs in translational studies. |
|
This publication describes a novel biological method that selects human BMSCs based on NOTCH2 expression and activation of the NOTCH signaling pathway in cultured BMSCs via a tissue culture plate coated with recombinant human JAGGED1 (JAG1) ligand. We demonstrate that transient JAG1-mediated NOTCH signaling promotes human BMSC maintenance and expansion while increasing their skeletogenic differentiation capacity, both ex vivo and in vivo. This study is the first to describe a NOTCH-mediated methodology for maintaining and expanding human BMSCs. It will be a platform for future clinical or translational skeletal regeneration and repair studies. |
|
This volume, Skeletal Development and Repair, in the Methods in Molecular Biology series, is designed as a comprehensive laboratory manual for all levels of basic research scientists working in the broad fields of skeletal development and skeletal repair research. The protocols highlighted here encompass the most current and cutting-edge techniques in skeletal development and repair and showcase those protocols that have been modified and perfected over several decades of skeletal research. Three chapters from this work were developed directly by the Hilton Laboratory. |
|
This publication is the first to indicate that the RBP-Jκ–dependent Notch pathway is a novel pathway involved in joint maintenance and articular cartilage homeostasis, a critical regulator of articular cartilage ECM-related molecules, and a potentially important therapeutic target for OA-like joint disease. |
|
This publication was the first to demonstrate that Notch regulation of chondrocyte maturation is solely mediated via the RBPjκ-dependent pathway and that the perichondrium or osteogenic lineage influences chondrocyte terminal maturation. Furthermore, we identified the cartilage-specific RBPjκ-independent pathway as a critical regulator of chondrocyte proliferation, survival, and columnar chondrocyte organization. We also identified RBPjk-independent signals as an essential long-range cell non-autonomous regulator of perichondral bone formation that coordinates chondrocyte and osteoblast differentiation during skeletal development. |
|
This publication demonstrates for the first time that the RBPjkappa-dependent Notch signaling pathway is a crucial regulator of mesenchymal progenitor cell (MPC) proliferation and differentiation during skeletal development. We also implicated the Notch pathway as a general suppressor of MPC differentiation that does not bias lineage allocation. Finally, Hes1 was identified as an RBPjk-dependent Notch target gene essential for MPC maintenance and suppressing in vitro chondrogenesis. |
|
This publication was the first to demonstrate the importance of Notch signaling in bone marrow mesenchymal progenitor cells. Our data support a model wherein Notch signaling in bone marrow typically maintains a pool of mesenchymal progenitors by suppressing osteoblast differentiation. Thus, mesenchymal progenitors may be expanded in vitro by activating the Notch pathway, bone formation in vivo may be enhanced by transiently suppressing this pathway. |
Research Opportunities
Postdoctoral Researchers, Graduate/Medical Students, and Technicians interested in Musculoskeletal Development, Disease, and Repair Research
The Hilton Laboratory within the Duke Orthopaedic Cellular, Developmental, and Genome Laboratories at Duke University School of Medicine utilizes novel mouse genetic and surgical models to study musculoskeletal development, disease, and repair/regeneration. Our research particularly emphasizes identifying underlying molecular mechanisms that contribute to normal musculoskeletal development and repair processes or disease pathogenesis. One of the major focuses of the Hilton Lab is to utilize conditional (Cre/LoxP) mutant and transgenic mouse models coupled with primary cell culture and biochemistry to understand the contributions of various signaling pathways to the development and maintenance of the growth plate and joint cartilages, as well as, to musculoskeletal development, repair, and regeneration. To learn more about the Hilton Laboratory and their research, please send an email to:
Matthew J. Hilton, PhD
matthew.hilton@duke.edu
News and Events
To learn more about the Hilton Laboratory and its research, please email Matthew J. Hilton, PhD.